Formulators of curable acrylic materials are challenged with brittleness. This inherent limitation of acrylic chemistry creates the need for new toughening solutions. Formally, the term toughness refers to the ability of a material to absorb energy or stress and plastically deform without fracturing. It is represented as the area under a tensile stress-strain curve.
An acrylic part can experience stress from external sources such as bending, twisting, compression, or stretching. The stress can also come from internal forces, such as shrinkage stress during polymerization or from a mismatch in thermal expansion coefficients of blended materials.
A number of toughening approaches already exist today. One effective strategy is to formulate with core-shell toughening agents. Unfortunately, the core-shell approach is limited to manufacturing operations that are equipped to handle powders and disperse them fully.
A new solution has been developed in the form of pre-dispersed masterbatches of core-shell particles in (meth)acrylate monomers. These new liquid toughener dispersions are stable and have been proven to increase the toughness of acrylic materials. The utility of these tougheners has been demonstrated in light-curable thermoset formulations and in 2K acrylic structural adhesives.
Core-Shell Toughening without Handling Powder
Core-shell manufacturers optimize the size of the primary particles in order to achieve the desired toughening effect. The toughening can occur via one or more mechanisms, such as energy absorption by the rubber, debonding at the particle-matrix interface, matrix crazing, and shear yielding. Agglomerations of particles, on the other hand, act as stress concentrators due to their larger size. In other words, agglomerations have the opposite effect on performance.
Toughening of a material with core-shell particles requires that the particles are fully dispersed in the polymer continuous phase. Naturally, this morphology requires a successful dispersion of core-shell particles in the resin composition prior to polymerization. A key aspect of using core-shell particles in light- and peroxide-curable compositions is ensuring that high dispersion quality.
Achieving high dispersion quality usually requires aggressive mixing or milling of the powder into the resin. Examples of suitable equipment include a double-planetary mixer, rotor stator, high-speed dispersion bade, or a three-roll mill. The mixing energy needed is high and varies with the compatibility between the particles and the liquid resin. Ultimately, manufacturing operations that have the equipment and the safety precautions in place are satisfied with using powder additives. For others, core-shell particles are not an option.
The liquid toughener dispersions presented here enable formulators to enjoy the benefits of core-shell toughening when they previously could not. These tougheners are particularly useful as ingredients in acrylic structural adhesives and other thermoset systems. The key to this strategy is that the core-shell particles are already fully dispersed in the liquid resin. That high dispersion quality is transferred to the final product during formulating. For demonstration, the ease-of-use of the liquid toughener dispersions were compared experimentally to the addition of core-shell powder alone.
Two analogous adhesive compositions were formulated: one with traditional core-shell particles and the other with the liquid masterbatch approach. The traditional core-shell toughener was a commercially available product that has been on the market for decades. A dispersion of 30% proprietary MBS core-shell particles in 70% phenoxyethyl methacrylate (w/w%), referred to here as AR340Y30, served as the exemplary liquid toughener.
The adhesive compositions were formulated in-house based on a typical B-side formulation for a 2K structural adhesive. At the end, both adhesives had identical loadings of core-shell particles and identical loadings of monomer. Table 1 shows the final adhesive makeup.
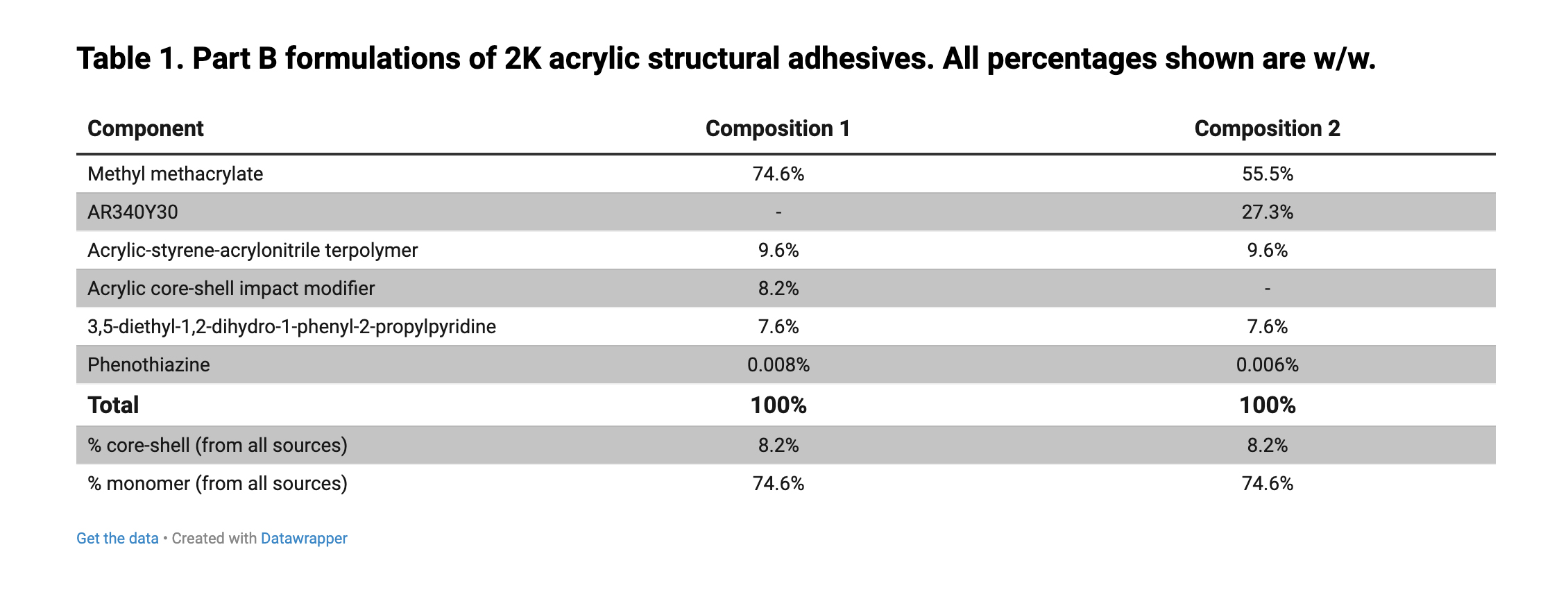
*Click the image for greater detail
The first step of the experiment was the preparation of homogenous pre-blends of the non-toughener ingredients. Once homogenous, the tougheners were added to the pre-blends and each was mixed with an overhead mixer. All aspects of the mixing (equipment used, shear rate, mixing duration, temperature, and so on) were identical for both mixes. During and after mixing, the dispersion quality was observed (see Figure 1).
Figure 1. Adhesive Compositions 1 (left) and 2 (right) after mixing at r.t. at 400 rpm for 20 min.
*Click the image for greater detail
The results were clear: higher dispersion quality was obtained using the pre-dispersed masterbatch approach than with direct powder addition. The finished adhesive comprising AR340Y30 was visibly smooth flowing, low viscosity, and homogenous. By comparison, the adhesive comprising the core-shell powder was higher viscosity and grainy. Other follow-up experiments demonstrated that in order to achieve identical dispersion quality (e.g., as measured with a Hegman gauge), much longer mixing times are required for the powder than for AR340Y30.
Evaluation in Simple Curable Systems
After the ease-of-use was demonstrated, the remaining experiments evaluated the effectiveness of this approach at increasing toughness. This work consisted of two investigations. For the first, tougheners were evaluated in simple thermoset formulations consisting mainly of the toughener and acrylic oligomer (to thicken the system). Once cured, the materials were subject to a number of tests relating to toughness. These tests included notched Izod impact, flexural modulus, Shore hardness, direct impact, and assessments of polymerization-induced shrinkage and cracking.
In all cases, samples comprising the new toughener outperformed untoughened controls. Some of the experiments were set up as ladder studies; in these cases, the performance increased with the loading of the liquid toughener dispersion. Overall, the results demonstrated that the liquid core-shell masterbatch approach is a practical way for formulators of acrylic materials to improve performance.
Each experiment in this investigation used curable compositions comprising either the AR340Y30 toughener or another referred to as AR210Y38. AR210Y38 is a dispersion of 38% proprietary MBS core-shell particles in 62% PEG200 dimethacrylate (w/w). The same proprietary core-shell particles are used in both masterbatches.
Table 2 shows the compositions formulated with these tougheners, along with the control compositions having only the corresponding methacrylate monomers. In these experiments, AR210Y38 was used in the photocurable systems and AR340Y30 for thermal curing. That said, they are mutually suitable for both photocure and thermal cure applications.
*Click the image for greater detail
Shrinkage
These liquid toughener dispersions provide formulators with a strategy to reduce shrinkage. Shrinkage incurred from polymerization can be a major issue for nearly all applications. Shrinkage occurs during curing as the molecules are pulled closer together during the reaction. High levels of shrinkage can cause undesirable internal stresses, along with reduced adhesion to substrate material.
In this experiment, the specific gravities of Compositions 2-6 were measured before and after photopolymerization at room temperature. Shrinkage incurred during curing was then calculated from the change in specific gravity (ρ):
% shrinkage = (ρcured - ρliquid)/ρcured × 100
The results of this experiment are shown in Figure 2. Across the four formulations, shrinkage decreased with the toughener loading. At 50% of the total composition, AR210Y38 decreased the shrinkage from 8.3% to 5.9%, a nearly 30% improvement. This reduction should help improve adhesion, reduce curl on coated flexible substrates, reduce internal stresses in bulk substrates, and deliver many other positive benefits to end-use products.
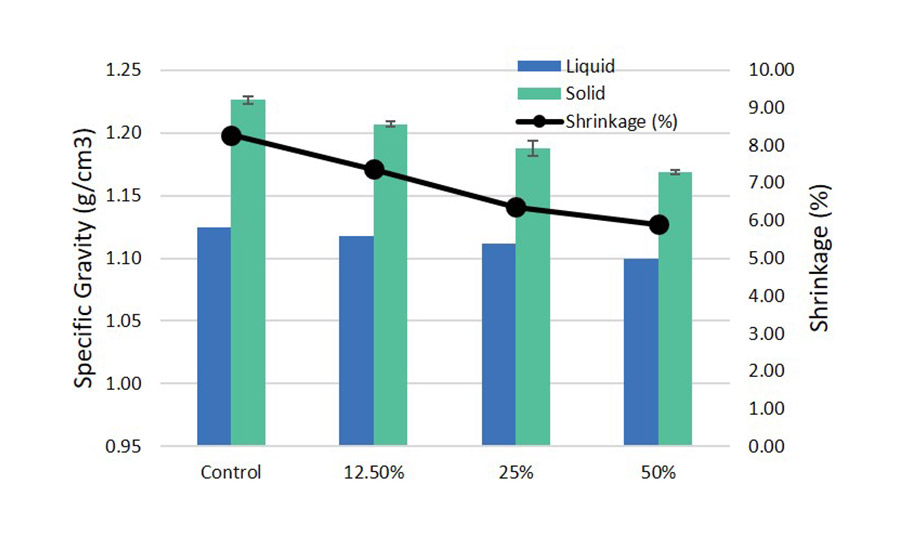
*Click the image for greater detail
Bulk Crack Performance
This study demonstrated that formulating with the new tougheners reduced bulk cracking and delamination during curing. These unfortunate phenomena are extreme symptoms of shrinkage stress. Cracking can manifest from high reaction temperatures, increased levels of shrinkage, and internal stresses imparted during curing.
In this experiment, curable compositions were made with and without the new toughener and cured in metal weighing dishes. After curing, the samples were visually inspected for cracks and delamination. As anticipated, the compositions containing the new tougheners were less prone to these problems.
In this experiment, a urethane methacrylate oligomer, polyethylene glycol dimethacrylate monomer, and AR210Y38 were mixed together in a reactor with mechanical stirring with four parts by weight of a peroxyester and 0.5 parts by weight of an amine accelerator. The formulations were blended for 30 min at room temperature. The resultant mixtures were poured in a metallic cup having a diameter of 5 cm and left to cure at room temperature for 10 min.
Once the reaction was finished, a solid material having a diameter of 5 cm and a thickness of 3.5 cm was removed from the mold and cleaned with acetone. Pictures of the resultant cured materials are shown in Figure 3. The untoughened sample showed significant signs of cracking. The sample with AR210Y38 at 12.5 wt% showed no signs of imperfections.
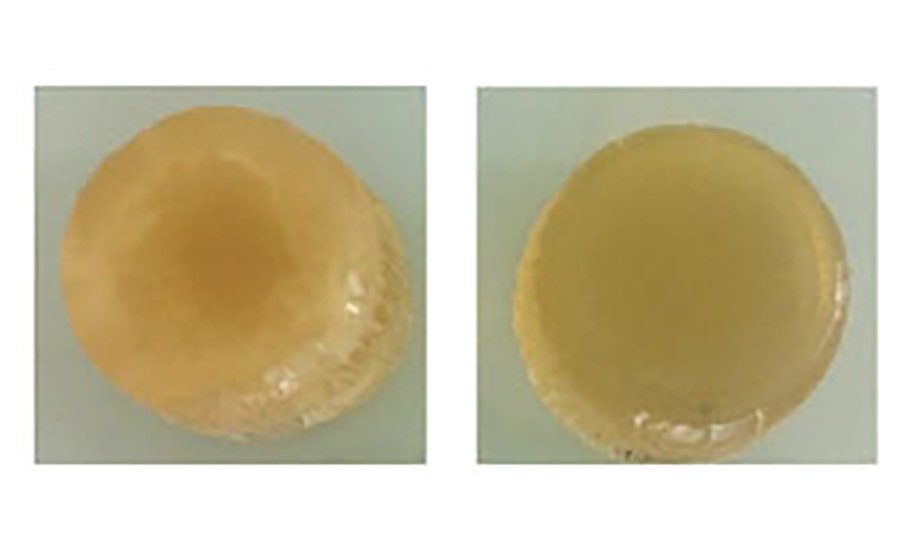
Figure 3. Blends without core-shell particles (left) and with AR210Y38 (right) bulk cured to observe differences in polymerization-induced cracking.
*Click the image for greater detail
Hardness and Flexural Modulus
This experiment demonstrated that the new tougheners make materials deform more easily (i.e., with less force) without decreasing the hardness. The hardness was measured with a standard durometer. The force to flex the material was quantified by measuring the flexural modulus according to ASTM D790. Flexural modulus is related to the amount of energy required to bend a substrate. The higher the modulus, the more energy is required to bend the substrate.
In this experiment, Compositions 3-10 were cured and subjected to the hardness and flexural testing. Figure 4 shows the measured flexural modulus and hardness as a function of the amount of AR210Y38 included in both model systems.
Figure 4. Shore D hardness and flexural modulus of test materials. The left plot corresponds to Compositions 3-6 and the right plot to Compositions 7-10. Error bars are 1 standard deviation.
*Click the image for greater detail
In both cases, the flexural moduli decreased with increased loadings of AR210Y38. In other words, the more toughener, the easier it was to bend. The epoxy system reduced by 27% while the urethane system reduced by 38% when loadings were increased to 50 wt% AR210Y38.
The hardness testing, on the other hand, showed that this improvement in flexibility does not come at a steep cost. Specifically, as the amount of AR210Y38 loading increased, the hardness of the cured samples decreased only slightly.
Notched Izod Impact
This experiment established that the liquid toughener dispersions improve the impact resistance of cured acrylic materials. Notched Izod impact was performed in accordance with ASTM D256. Figure 5 shows the Izod impact results for Compositions 3-12.
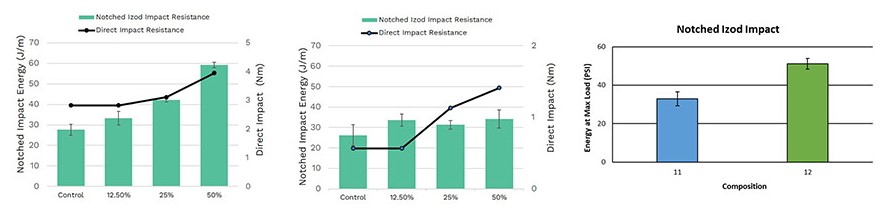
Figure 5. Notched Izod impact resistance and direct impact performance of test materials. The left plot corresponds to Compositions 7-10, the center to Compositions 3-6, and the right to Compositions 11 and 12. Error bars are 1 standard deviation.
*Click the image for greater detail
These compositions spanned a wide range of formulations, including both liquid toughener dispersions, three oligomer chemistries, and multiple toughener loadings. Across the board, the masterbatch tougheners produced samples with higher impact resistance than the controls. AR210Y38 in a urethane system (i.e., Compositions 7-10) showed a particularly large increase in impact resistance, even at only 12.5%.
Direct Impact
Direct impact was evaluated by striking bars of cured materials with a 1 kg weighted dart from various heights. The method was a modified version of ASTM D2794. After impact, the bars were inspected for visible signs of duress.
In a successful test, the bars remained whole and displayed no significant visible defects after impact. Figure 5 shows an overlay of both notched impact and direct impact results. The urethane system showed significant improvement with increased loadings up through 50 wt% AR210Y38.
Unlike what was observed in the notched Izod results, in the direct impact results, samples using AR210Y38 showed no significant direct impact improvement over the control at 12.50 wt%. There was however, a large improvement when loadings were increased to 25 wt% of AR210Y38.
Case Study in 2K Acrylic Structural Adhesives
A case study evaluated the effectiveness of the new toughening approach in MMA-based 2K structural adhesives. Adhesives comprising AR340Y30 were evaluated against untoughened controls, as well as against adhesives comprising the monomer or core-shell components of AR340Y30 alone. Ultimately, AR340Y30 was shown to substantially improve lap shear on steel and boost tensile toughness.
Acrylic structural adhesives were chosen for the case study application due to their increasing prevalence in manufacturing. Contributing to this trend are their benefits over mechanical fasteners such as efficient application, low final weight, and longevity. Automotive applications, for example, benefit highly from the weight reduction afforded by structural adhesives.
The adhesives used for these experiments are shown in Table 3. All adhesives that had core-shell toughener had identical core-shell loadings, regardless of whether the core-shell particles were introduced in powder or liquid masterbatch form. Further, all five adhesives had the same amount of monomer. To achieve this constancy, the amount of MMA was adjusted according to the amount of phenoxyethyl methacrylate monomer present.
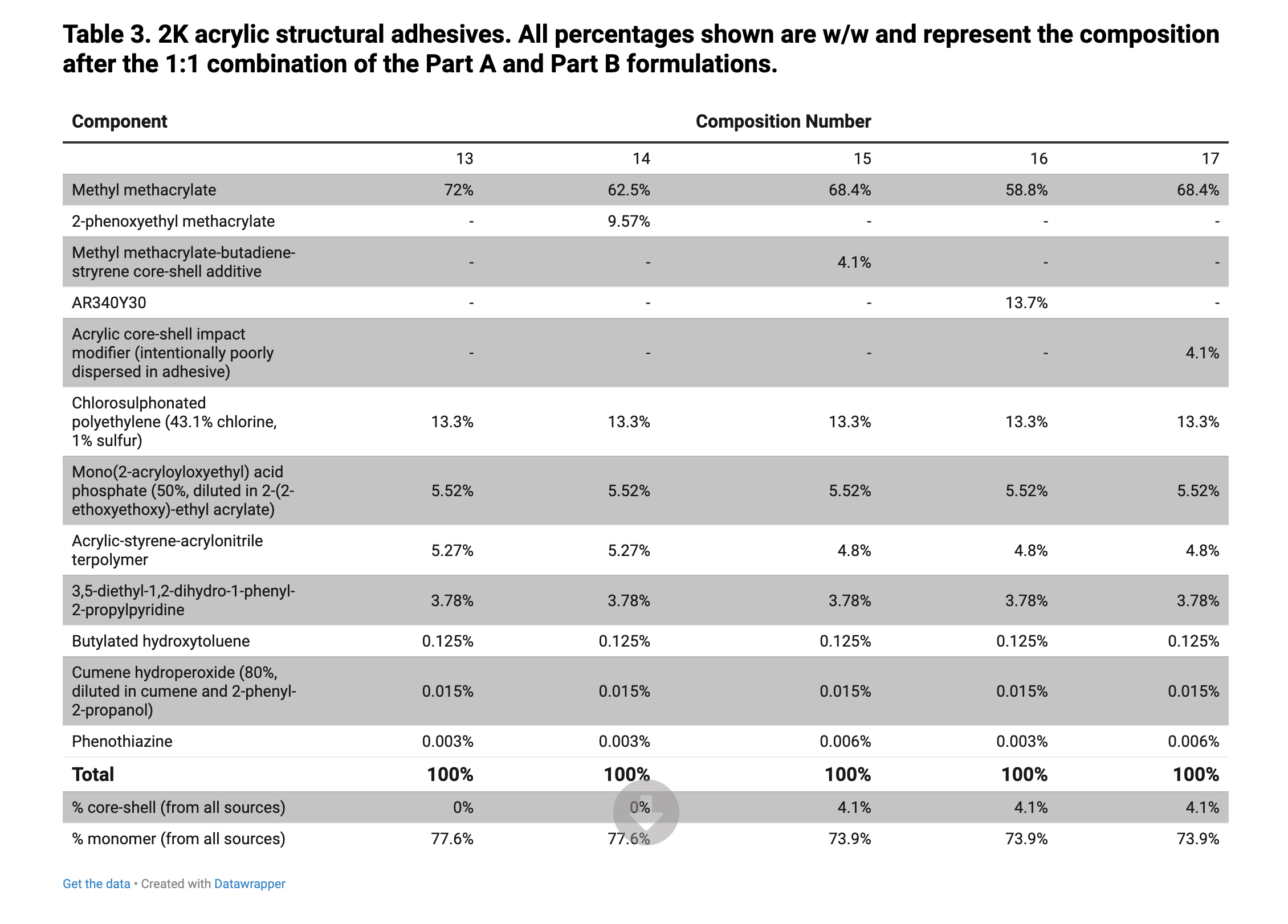
*Click the image for greater detail
Tensile Testing
This study evaluated the effects of AR340Y30 on the tensile properties of the adhesives. Tensile testing allows for quantification of toughness itself, as well as strength and elongation separately. With tensile testing, we can also quantify the modulus of the material. The key result from this experiment is that AR340Y30 substantially increases toughness with only slight cost to the modulus.
The results of the tensile testing are shown in Figure 6. The increase in elongation produced by AR340Y30 in combination with a nearly unchanged tensile strength explains the increase in toughness.
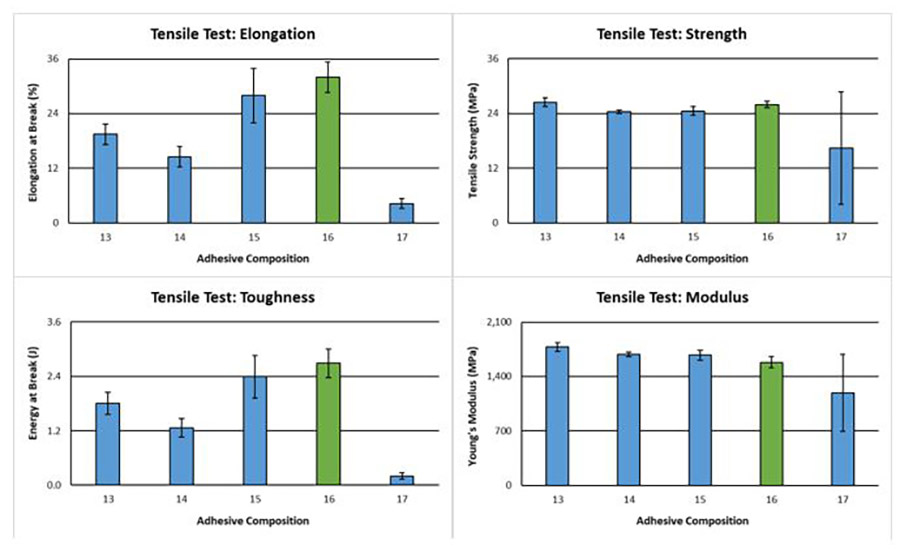
With many toughening approaches, modulus and toughness exhibit an inconvenient tradeoff. Figure 6 shows that formulators need not compromise modulus with the new toughener. The results for Compositions 14 and 15 suggest that the higher toughness is produced by the core-shell component of AR340Y30 rather than the monomer component.
Lastly, the importance of good dispersion quality are evident in the poor performance of Composition 17. Qualitative observations align with the measurements. Microscopic core-shell agglomerations in Composition 17 produced visible stress concentration (i.e., uneven whitening) during the tensile testing. By comparison, all other samples exhibited even whitening over the narrow region of the specimens during testing, as is characteristic of a uniform dispersed phase.
Lap Shear
This study evaluated the effects of AR340Y30 on the lap shear strength of the adhesives using both stainless steel and polypropylene substrates. Lap shear testing can measure one of two properties, depending on how the adhesive breaks during the test. If the adhesive fails adhesively, the measurement corresponds to the strength of the bond at the interface of the adhesive layer and the substrate material. If the adhesive fails cohesively, on the other hand, the measurement corresponds to the cohesive strength of the material.
All adhesives in this study failed cohesively on stainless steel and adhesively on polypropylene. The key result of the lap shear testing is that AR340Y30 substantially increases the lap shear strength on stainless steel.
The lap shear results are summarized in Figure 7. The left plot shows the large improvement in lap shear strength vs. toughener level on stainless steel. On plastic, there was a reduction to lap shear strength on polypropylene, although this reduction is comparably minor. The results for Compositions 14 and 15 suggest that the lap shear improvement associated with AR340Y30 is not obtained from using either of its components isolated from the other. Lastly, poor dispersion of Composition 17 was shown to have less of an influence on the lap shear results than the tensile properties.
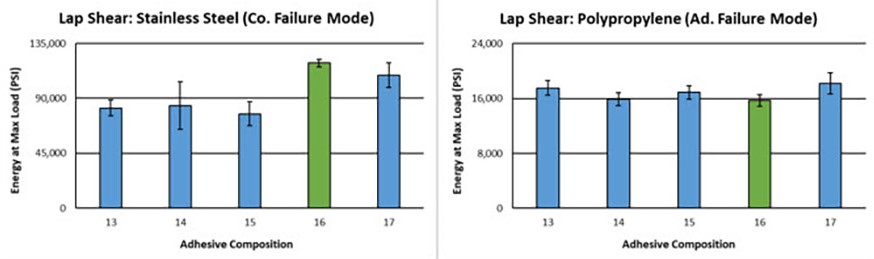
Figure 7. Lap shear test results for 2K acrylic structural adhesives. Stainless steel lap shear tested in accordance with ASTM D1002. The method was then adapted for polypropylene substrate. Error bars are 80% confidence intervals.
Conclusions
A new toughening approach was investigated as a strategy to enable core-shell toughening while maintaining the benefits of liquid handling. These new liquid toughener dispersions offer all of the benefits associated with core-shell impact modifications, including improved notched Izod impact, flexural modulus, direct impact, tensile properties, lap shear on stainless steel, and less polymerization-induced shrinkage and cracking, without handling and dispersing powders.
Importantly, these benefits do not force formulators to compromise other properties like modulus, hardness, or adhesion to plastics. The masterbatch approach will expand the use of core-shell toughening to applications and operations where it was previously not feasible.
For additional information, contact George Fortman at george.fortman@arkema.com or visit www.arkema.com.
Authors’ note: The authors would like to thank the following researchers at Arkema for their contribution to this research: Linda Franceschini, Sanskaar Gupta, Anthony Campagna, and Liza Marasinghe.
Note: Opening image courtesy of AnuchaCheechang via GettyImages.com.