
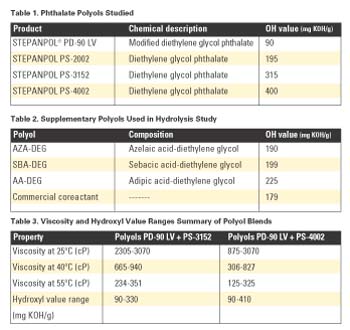
In two-component adhesive systems, it is often imperative that the individual materials of the polyol side remain soluble to avoid separation after mixing and prior to use. Such solubility requirements may restrict the formulation latitude to components that are either soluble in one another or contain a cosolvent to maintain compatibility. While polyether and polyester polyols may each possess a unique set of benefits for an adhesive system, they are often insoluble in one another, creating barriers to simultaneously exploiting the advantages of both types of polyols. A workhorse, or "base," polyol, compatible with either polyether or polyester polyols, would therefore offer the development chemist a broader formulating latitude to meet the diverse demands of various FlexPack markets.
Diethylene glycol phthalates, esters of phthalic anhydride and diethylene glycol, have demonstrated unique bonding advantages to various types of substrates.1-3 In addition to their utility in adhesives, we have shown their superior resistance to degradation by water (or hydrolytic attack) in acidic conditions.4 However, these esters are incompatible with polypropylene glycols, a common trend with polyester polyols in general. Further, the relationship between the viscosity of diethylene glycol phthalates and molecular weight is approximately exponential, with a 1,000 molecular weight diethylene glycol-phthalate having a 25°C dynamic viscosity of approximately 200,000 cP and a 2,000 MW analogue exhibiting a viscosity over 3,000,000 at the same temperature.
To preserve the advantages of hydrolysis resistance and good bonding characteristics, while offering higher (>1,000 g/mole) molecular weight phthalates with manageable viscosities (<3,000 cP at 25°C) and a range of compatibility with polyethers and polyesters, we have developed a series of low viscosity ("LV") phthalate esters using a proprietary technology. One product, STEPANPOL® PD-90 LV, is a nominal 90 mg KOH/g hydroxyl value diol (or 1,247 g/mole, calculated) with a typical room temperature viscosity under 2,500 cP. In this work, we investigated the behavior of solventless two-component polyurethane adhesives prepared from blends of PD-90 LV, with each of two other diethylene glycol phthalates as coreactants (see Table 1).
Specifically, various blends of PD-90 LV with PS-3152 or PS-4002 were measured for viscosity and inspected for visual compatibility; the viscosity data was analyzed, modeled, and mapped as a designed experiment using Design-Expert software, version 6.0.6 (Stat-Ease, Minneapolis).
Our investigation of the resistance of phthalate esters to hydrolysis was extended here from previous work4 to provide direct comparisons of the polyols in Table 1 and esters commonly used in FlexPack polyurethane adhesives. Additional polyols used in this study are shown in Table 2.
Laminates of aluminum foil to either treated biaxial oriented polypropylene (BOPP), linear low-density polyethylene (LLDPE) or polyethylene terephthalate (PET) films were made by hand using blends of polyols from Table 1 as coreactants and a commercially available prepolymer as an isocyanate. Peel strengths of these laminates were compared to a commercial standard prepared in the same manner using a modified version of ASTM Method D 1876-95.
Experimental Details
GPC Analysis for Hydrolysis StudiesGel Permeation Chromatography (GPC) analysis was performed on a system using equipment and software from Waters Corp. The company's Millennium 32 GPC software provided peak information (e.g., retention times and area counts).
The GPC system used in this work consisted of the following elements. THF (solvent grade material from VWR Scientific, stabilized with 250 ppm BHT) was used as the mobile phase at a 1.0 ml/min solvent flow rate delivered by a Waters model 515 dual-piston solvent pump. The samples were then injected by a Waters 717+ autosampler onto a column bank. The bank was held at 45

Results and Discussion
Viscosity and SolubilityPlots of viscosity as a function of composition and temperature for blends of PD-90 LV + PS-3152 and PD-90 LV + PS-4002 are shown in Figure 1. Hydroxyl values are used as the x-axis to indicate composition.
The contour lines in the graphs represent constant-viscosity mixtures. With the PD-90 LV + PS-3152 blend, the viscosities were typically higher than the PD-90 LV + PS-4002 blend at the same temperatures due to the inherently lower viscosity of PS-4002.
Coreactants (B-side) for flexible packaging adhesives are reacted with the isocyanate component (A-side), usually a prepolymer, to make a flexible packaging adhesive. The coreactants are single polyols or multiple-polyol blends. Typically, these adhesives are applied at temperatures between 40-70ºC, so the viscosities of both the coreactants and the isocyanates are important to processing these adhesives. Another significant factor is the hydroxyl value of the coreactant, as this determines the amount of isocyanate required.
All blends of PD-90 LV with PS-3152 or PS-4002 prepared for the DOE study appeared clear at 25, 40 and 55ºC. Also, clear mixtures were observed for 1:2, 1:1 and 2:1 weight blends of PD-90 LV and PPG-2000, showing PD-90 LV to be compatible with a standard polyether polyol as well as polyester polyols.

We previously reported on the hydrolysis of ortho-phthalate polyols, showing their superior hydrolytic stability compared to similar molecular weight polyols made from either aliphatic acids or polycaprolactones.4 Here, we extended this work to compare the hydrolytic stability of azelaic acid- and sebacic acid-based polyols to ortho-phthalate polyols, and to compare the hydrolysis of ortho-phthalate polyols in a basic environment to adipic acid-based polyol.
Separately, 2% water by weight was added to the AZA-DEG, SBA-DEG and PS-2002 polyols; acid values of these solutions were monitored at 95°C over time. Figure 2 shows the results of these tests.


The GPC traces for the ortho-phthalate polyol are practically identical; no additional peaks are seen. From these results, it is apparent that the ortho-phthalate polyols are more hydrolytically stable than both the AZA-DEG and SBA-DEG polyols commonly used in flexible packaging adhesives. Presumably, this is due to the inherent tendency of the ortho position of the esters to hinder hydrolysis.4



We previously used molecular modeling calculations to fundamentally explore why ortho-phthalate polyols have better hydrolytic stability compared to aliphatic acid polyols in neutral or acidic conditions.4 We now use a similar strategy to understand the hydrolytic stability of ortho-phthalate polyols in the presence of a strong base.
During ester hydrolysis, nucleophilic attack occurs on the carbonyl portion of an ester. Electrons from the nucleophile enter the lowest unoccupied molecular orbital (LUMO) as hydrolysis begins. Accordingly, the susceptibility to nucleophilic attack of a carbonyl portion of a molecule may be understood by analyzing the LUMO characteristics of an optimized conformation. Two molecular structures, an adipic acid-diethylene glycol diester (AA-DEG) and an ortho-phthalate diethylene glycol diester (OP-DEG), were optimized by semi-empirical methods using a conventional PM3 modeling algorithm.5
Figure 7a is a representation of an optimized structure with LUMO superimposition of the AA-DEG diester; the red and blue areas represent the LUMO. In Figure 7b, the electrostatic potential is mapped onto an isodensity surface that allows quantification of locations where nucleophilic attack would occur. Blue areas represent regions where nucleophilic attack is most likely to occur. The modeling program also allows estimation of the electrostatic potentials on these surfaces. The higher the number, the higher the susceptibility for nucleophilic attack. For the AA-DEG diester, this value was 0.179 kcal/mol at the carbonyl region of the ester.


This study evaluated several coreactant mixtures using PD-90 LV, PS-3152 and PS-4002. These blends were made at room temperature and then characterized. All blends did not separate and were clear. A commercial prepolymer was used in all of these laminates and had 16% NCO content. Table 4 shows the various properties of each mixture.



As with the Al and BOPP results above, Table 7 shows increasing maximum peel strength with increasing amounts of PS-3152 or PS-4002.
From these studies, it is clear that coreactant mixtures of PD-90 LV with either PS-3152 or PS-4002 provided comparable -or at times superior- performance to a commercial coreactant product.

Conclusion
In this study we demonstrated the versatile compatibility of a unique polyester polyol, PD-90 LV, with both polyester and polyether polyols. The temperature- and composition-dependency of viscosity of two series of blends were also mapped to enable chemists to match viscosity and formulation requirements.Hydrolysis resistance of ortho-phthalate polyols was also demonstrated under acidic and basic conditions and was found to be superior to azelaic and sebacic acid-based polyols. GPC was used to confirm hydrolysis phenomena under both basic and acidic environments. Molecular modeling provided a rationalization for the remarkable resistance to base hydrolysis of the phthalates.
Laboratory preparations also showed ortho-phthalate polyols to give satisfactory bonding results between aluminum and TEP, BOPP and LLDPE films.
As a workhorse polyol, PD-90 LV, when used with either PS-3152 or PS-4002, gave a coreactant system that offered bonding characteristics comparable to a commercial system. Additionally, PD-90 LV offered the benefits of compatibility with polyesters and a polyether polyol , as well as a superior hydrolysis resistance not generally observed with conventional polyester polyols.
For more information, contact Stepan Co., 22 W. Frontage Road, Northfield, IL 60093; phone (847) 446-7500; fax (847) 501-2466; or e-mail mobrien@stepan.com .
STEPANPOL® is a registered trademark of the Stepan Co.
This article is based on a paper presented at the Fall 2003 ASC Meeting in Cleveland.
SIDEBAR: Abbreviations
PU: PolyurethaneOHV: Hydroxyl value
AZA-DEG: Azelaic acid-diethylene glycol polyester polyol
SBA-DEG: Sebacic acid-diethylene glycol polyester polyol
PET: Polyethylene terephthalate
BOPP: Oriented polypropylene
LLDPE: Linear low-density polyethylene
AA-DEG: Adipic acid-diethylene glycol polyester polyol
OP-DEG: Ortho-phthalate diethylene glycol polyester polyol